Roll forming is a process that shapes metal sheets into specific forms through mechanical means. Common applications include the production of circular and conical components. However, the potential of roll forming technology goes far beyond these — through precise mechanical design and process optimization, it can also efficiently produce angular metal structures such as rectangular and square components.
Modern roll forming equipment is now integrated with crowning systems, intelligent software, and high-precision controllers, enabling forming accuracy at the millimeter or even micrometer level, and ensuring stable and consistent product quality.
This flexible and multifunctional sheet metal processing system has become one of the core pieces of equipment in modern manufacturing. From metal housings of household appliances to precision components for aerospace, roll forming technology is widely used across various manufacturing scenarios, thanks to its efficiency, precision, and high degree of customization.
Despite the complexity and diversity of sheet metal bending techniques, roll forming (specifically referring to the sheet metal rolling process in this context) always follows a unique forming logic. This article will focus on the principles and applications of roll forming technology.
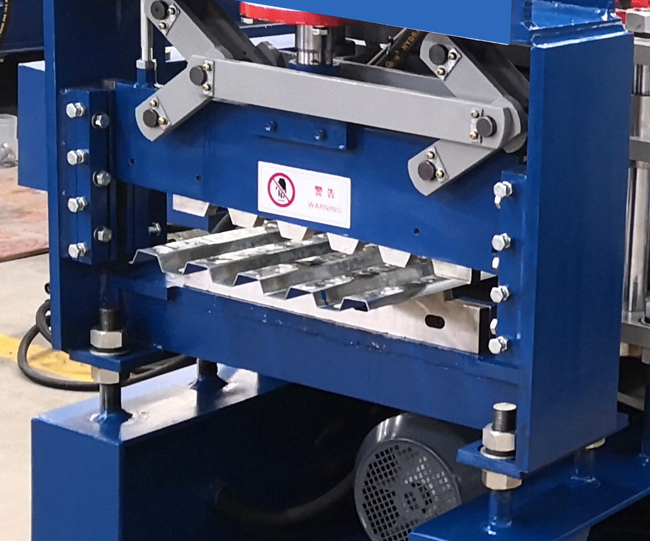
Working principle of roll forming machine
Roll forming technology uses specific mechanical devices and process flows to gradually bend metal sheets into preset geometric shapes. Common products include circular (O-shaped), grooved (U-shaped) and angled (angled) structural parts. Its core working principle is to use the relative movement between rollers to apply controllable bending force to the material to cause plastic deformation.
Traditional plate rolling machines are usually equipped with upper and lower rollers as the basic structure. The upper roller is responsible for the clamping and positioning function, fixing the material through pressure and ensuring its stable transportation; the lower roller is used as an active drive unit to generate torque through rotational movement to push the material to bend continuously along the roller surface. With the evolution of technology, modern plate rolling machines have developed a variety of configurations with two rollers, three rollers and even four rollers, and their mechanical structure and motion logic have also been optimized to meet the needs of different material thicknesses, bending radii and production efficiency.
During the forming process, the metal sheet needs to undergo multiple cycles of bending between the rollers until it reaches the target shape. For complex details (such as edge fillets) or special bending requirements, auxiliary processes or special equipment are usually required for supplementary processing. It should be noted that the dimensional accuracy and shape stability of the final product are affected by multiple factors, including material properties (such as elastic modulus, yield strength), plate thickness, roller gap and pressure distribution, etc., which need to be dynamically adjusted through precise calculation and real-time monitoring.
This technology has become a core processing method in the fields of automobile manufacturing, building steel structure, shipbuilding, etc. due to its high efficiency, flexibility and cost advantages, and has shown significant economic benefits in large-scale production.
Roll Bending Machine System
Roll bending machines can primarily be categorized into mechanical and hydraulic types based on their driving methods. For details on the classification of roll bending machines by structural design (such as the number of rollers), you can refer to our previous specialized article.
Mechanical Three-Roller Roll Bending Machine
Mechanical three-roller roll bending machines can be divided into symmetric and asymmetric types based on the roller arrangement.
Symmetric Design:
Two parallel rollers are positioned at the bottom, with a third roller placed vertically and centrally above them. The bottom rollers provide the main driving force by rotating, which drives the material to continuously bend between the rollers. This is suitable for forming conventional cylindrical or conical workpieces.Asymmetric Design:
The upper roller is the main driving roller, positioned vertically in the center. A parallel roller is placed below it, and the third roller is offset laterally to the side. The side roller plays a key role in the pre-bending stage, allowing precise bending of the sheet edges, which typically requires auxiliary devices to achieve similar results in symmetric designs.
Hydraulic Roll Bending Machine
The upper roller of hydraulic roll bending machines is designed in a drum shape and uses a hydraulic system to achieve vertical lifting. This feature significantly improves the machine’s adaptability to different material thicknesses and ensures the straightness of the material’s edges. The flexibility of hydraulic drive and adjustment makes it more advantageous for handling complex shapes or high-precision requirements.
Both types of roll bending machines have their respective advantages in different applications: mechanical roll bending machines are known for their simple structure and easy maintenance, making them suitable for large-scale standardized production; hydraulic roll bending machines, with high precision control and flexibility, are more suitable for custom processing or industries that demand stringent surface quality.
Differences Between Cold Bending and Hot Bending in Terms of Process
In the field of sheet metal bending, there are various forming processes to choose from. However, before finalizing the specific rolling plan for a workpiece, it is essential to first distinguish between the two core process types: hot bending and cold bending.
Cold Bending Process
Cold bending refers to the direct bending process without the need for preheating the material. The key advantages of cold bending include high processing efficiency (no need for heating/cooling cycles) and low cost (no energy consumption or oxidation loss), making it particularly suitable for the regular forming of thin and medium-thick plates. However, due to the limited plasticity of the material at room temperature, cold bending requires high equipment rigidity and forming accuracy, and it is difficult to process high-strength materials or complex cross-sectional workpieces. If you plan to purchase a roll bending machine, you should comprehensively evaluate the applicability of the cold bending process based on product batch size, material properties, and precision requirements.
Hot Bending Process
Hot bending involves locally heating the workpiece above its recrystallization temperature, significantly enhancing the material’s plasticity, thus reducing forming resistance and improving dimensional accuracy. The technical advantages of hot bending include:
High Precision Forming: Hot bending can achieve stable processing of complex cross-sectional shapes and large curvature radius workpieces.
Wide Material Compatibility: Especially suitable for materials that are difficult to form through cold bending, such as high-strength steel, titanium alloys, and others.
Energy Savings Potential: Compared to cold bending, hot bending can reduce the power requirements of equipment drive systems. However, it is important to note that hot bending requires precise control of heating temperature, holding time, and cooling rate to prevent material performance degradation or surface oxidation.
Warm Bending Process (Hybrid Process)
Warm bending is a compromise between cold bending and hot bending. The workpiece is heated to a mid-temperature range (typically below the recrystallization temperature), which reduces forming resistance while minimizing the heat-affected zone. The advantages of warm bending include:
Better Formability: Reduces springback and improves dimensional stability.
Reduced Internal Stress: Compared to cold bending, residual internal stresses in the material are significantly reduced.
Moderate Equipment Cost: Does not require a full high-temperature environment, thus lowering the heat resistance requirements of the equipment. However, warm bending must balance heating energy consumption with forming efficiency and may experience local performance differences due to temperature gradients.
Selection Recommendations
Cold Bending: Suitable for thin plates, batch production, and workpieces with lower precision requirements.
Hot Bending: Best for high-value-added, complex cross-sectional, or difficult-to-process materials.
Warm Bending: Ideal for scenarios requiring high dimensional precision and material performance.
It is recommended to comprehensively evaluate the feasibility of the process based on the specific workpiece material, cross-sectional shape, production batch, and cost budget, combined with the technical support capabilities of the equipment supplier.
Analysis of Pre-Bending Process
In metal sheet processing, the bending pressure is applied only to the contact area of the rollers, which means that most of the “effective length” of the material does not undergo deformation. The undeformed part is referred to as the “straight edge.” The presence of a straight edge can cause the following issues:
Shrinkage and Deformation: The straight edge is prone to rebound or warping during subsequent processing, affecting the accuracy of the workpiece.
Material Waste: The straight edge area cannot be effectively utilized, leading to increased material waste.
Pre-bending, as a key preliminary process, can significantly reduce the risks of shrinkage and deformation in subsequent processing by locally plastically deforming the straight edge in advance. The core principle is to control the amount of pre-deformation, creating a transition zone between the bending area and the straight edge area, thereby balancing the stress distribution.
Theoretical Foundation of the Pre-Bending Process
Pre-bending parameters (such as pre-bending angle and pressure) need to be precisely calculated based on the bending type (symmetrical/unsymmetrical) and the sheet thickness.
Symmetrical Bending: The length of the straight edge is usually positively correlated with the sheet thickness, and the pre-bending amount is determined through formula derivation or empirical values.
Unsymmetrical Bending: Due to uneven forces, the straight edge area is prone to displacement, and pre-bending parameters need to be calculated by compensating for lateral forces.
Implementation Methods of the Pre-Bending Process
Based on process requirements, the operator can choose from the following technical solutions:
Press Bending: Vertical pressure is applied to the straight edge area using a mechanical press to achieve local plastic deformation.
Template Roll Bending: Custom molds combined with rollers are used to perform progressive bending of the straight edge.
Segment Roll Bending: A segmented roller structure is used to apply pressure in sections to pre-bend the straight edge.
Buffer Block Hydraulic Bending: Using hydraulic buffer devices to apply controllable liquid pressure to the straight edge, suitable for high-precision processing.
Key Control Points of the Pre-Bending Process
During the rolling process, it is necessary to ensure the precise centering of the workpiece to avoid the following issues:
Twisting: Misalignment of the workpiece can lead to cross-sectional distortion, affecting assembly accuracy.
Dimensional Deviation: Uneven lateral forces can cause fluctuations in the bending radius.
Methods for Centering Control:
Side Roller Centering: Dynamically adjust the position of the workpiece using side rollers.
Side Roller Grooving: Machining guiding grooves on the side roller surface to restrict lateral movement of the workpiece.
Inclined Centering: Using inclined rollers, gravity helps center the workpiece.
The pre-bending process optimizes the stress distribution in the straight edge area, significantly improving the forming accuracy and material utilization of metal sheets. In practical applications, it is necessary to comprehensively select the pre-bending method and parameter control strategies based on the workpiece structure, material properties, and equipment capabilities to achieve efficient and stable processing results.